Moving around on an unfamiliar celestial body
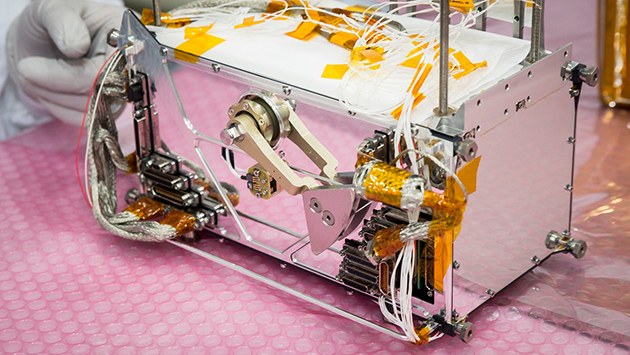
Moving around on small bodies is difficult, because the gravitational pull and thus the friction between a lander and the ground is very small. Conventional means of transport, such as wheels or chains, are based on traction and are thus unsuitable for use on asteroids. Therefore, a mobility mechanism was developed for MASCOT in order to enable movement in such an environment: the lander is equipped with a swing arm that accelerates and decelerates an eccentrically mounted mass. The resulting reaction forces, in turn, allow the lander to push itself away from the ground. As the gravitational pull is very low, even small push-off forces can result in big jumps: a distance of 17 metres can be achieved with an initial speed of only five centimetres per second. The gravitational acceleration on the surface of Ryugu is only 0.00015 metres per second squared and is thus 60.000 times lower than on Earth (9.81 m/s2).
Testing out this mechanism on Earth, however, proved very difficult, as the traditional methods for testing in microgravity conditions could not be put to any meaningful use. On parabolic flights, the gravity is zero on average, but there is also high-frequency acceleration, which, although low, nevertheless dominates any movement caused by the mobility. In addition, the acceleration on the MASCOT lander should not be brought down to zero, but must be precisely reduced to a very low value to mimic the conditions on Ryugu. For two-dimensional problems, it is common to use a pneumatic table for testing, which is based on the same principle as air hockey. Bodies can move freely across the plane of the table and rotate around the vertical axis. Gently tilting the table also introduces a slight force of gravity. However, the mechanism is designed to make MASCOT rotate around all three axes: as the accelerated mass is eccentrically mounted, it creates torques around all of the axes in addition to the rotating centrifugal force in the plane of the arm. The result is that, with every motion, MASCOT is moving and rotating in three directions, while at the same time shifting its centre of gravity. This also makes counterbalancing the weight force with a helium balloon difficult in tests conducted on Earth.
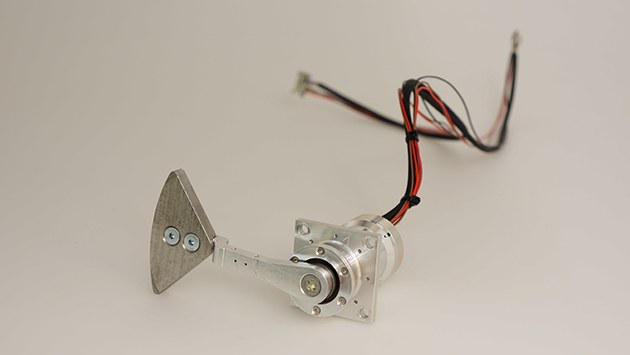
Predicting the lander's movements on the asteroid thus means resorting to numerical models and simulations. There are numerous models for simulating contact forces between bodies. Simulating the effect of the mobility mechanism means determining how MASCOT's geometry intersects with that of the ground at every point in time, and using the depth of the penetration to calculate a contact force based on a mass-spring-damper model. It is nevertheless difficult to establish parameters required by these models such as stiffness or the damping of the ground, as no data is available relating to the conditions on asteroids like Ryugu. Unfortunately, precise movement in microgravity is heavily dependent on such parameters, while the lander's mobility mechanism must be able to cope with both bare rock and porous, pebble-covered surfaces.
The problems faced by MASCOT were solved by calculating different motion profiles optimised for three different types of ground surface. The Autonomy Manager tests out each of these in succession by performing a manoeuvre. If a motion profile does not lead to the right outcome, an action suitable for a different type of ground surface is carried out. After each manoeuvre, distance sensors on the sides of the lander are used to check which side faces the ground. LEDs and photodiodes that measure the light reflected by the surrounding terrain are mounted on all sides. The stronger the measured signal, the closer the sensor is to an obstacle or the ground. In addition, MASCOT has photocells that measure the sunlight and further improve the attitude estimation. These data can also be used to evaluate the performance of the mobility. Also, pictures are taken before and after each manoeuvre, which can also be used to deduce the movement.
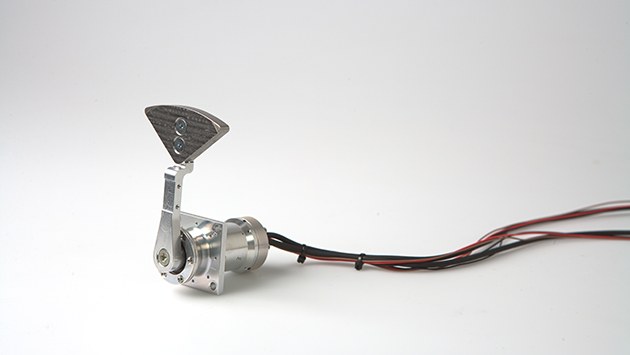
Nevertheless, controlled relocation and uprighting of a lander on a small body remains a challenge. When researching completely unfamiliar bodies, such as the asteroid Ryugu, it is very difficult to create a robust mechanism that can cope with the whole spectrum of potential environmental conditions. When MASCOT separates from the space probe Hayabusa2 on 3 October, falls onto the asteroid and begins working there autonomously using its four scientific instruments, we will finally know how it actually moves across the asteroid’s surface.
Tags: