Multi-phase Flow and alternative Fuels
DLR/NASA/Florian Friz
The research activities in the department focus on enabling an effective transition to sustainable aviation fuels (SAF). The department places particular emphasis on the holistic evaluation and optimization of sustainable fuels through fuel design and digital methods.
The aim is to develop sustainable fuels with a particular focus on minimizing the impact on the climate while enabling global mobility, cultural exchange and fostering economic growth in a world of tomorrow worth looking forward to.
To be used in an aircraft, any new production pathway for a synthetic blending component (SBC) must follow a rigorous qualification and approval procedure developed by ASTM International. This ASTM D4054 procedure makes it possible to define the production process, the specifications of the SBC and its maximum blending ratio with fossil jet fuel. The resulting Annex in ASTM D7566 ensures that the SBC and the blended fuel (SAF) meet all the required technical and safety criteria and hence can be used safely as a drop-in fuel in the existing infrastructure and aircrafts.
In this context, a wide variety of fuel properties must be tested and critically assessed. The process tends to be time-consuming and expensive, requiring substantial quantities of fuel that are often not readily available during the initial phases of developing a new fuel production process. Together with US partners of the University of Dayton and the Washington State University, our team has developed a pre-screening procedure before the official ASTM approval process. Based on low volumes (starting from a few milliliters) and probabilistic machine learning models we evaluate new fuel candidates with regard to the suitability for fuel approval.
Background
According to all roadmaps forecasting the path towards civil aviation climate neutrality, sustainable aviation fuel (SAF) plays the major role. This is no coincidence, as SAF can reduce the climate impact of aviation substantially, both in terms of CO2 and non-CO2 emissions. A major advantage is that these fuels can be readily utilized in existing and future aircraft engines, as well as with the current distribution networks. This is commonly referred to as the drop-in fuel concept.
Sustainable aviation fuels include biobased and non-biobased synthetic jet fuels, also known as Renewable Fuels from Non-Biological Origin (RNFBO's), obtained from CO2 captured from the atmosphere or from inevitable sources in industrial processes and renewable hydrogen. This category is often also referred to as e-fuels and Power to Liquid (PtL) fuels when the hydrogen is obtained by electrolysis of water using renewable electricity.
Although the demand for SAF is increasing through market and legislation, the introduction of these fuels is associated with major challenges for the aviation industry. On the one hand, it is desirable to approve as many SAFs as possible, also to widen the range of feedstocks. On the other hand, there is a need to ensure that the use of new fuels does not result in any risks. Ensuring safety is the topmost concern in aviation, i.e. all approved fuels are safe for flying.
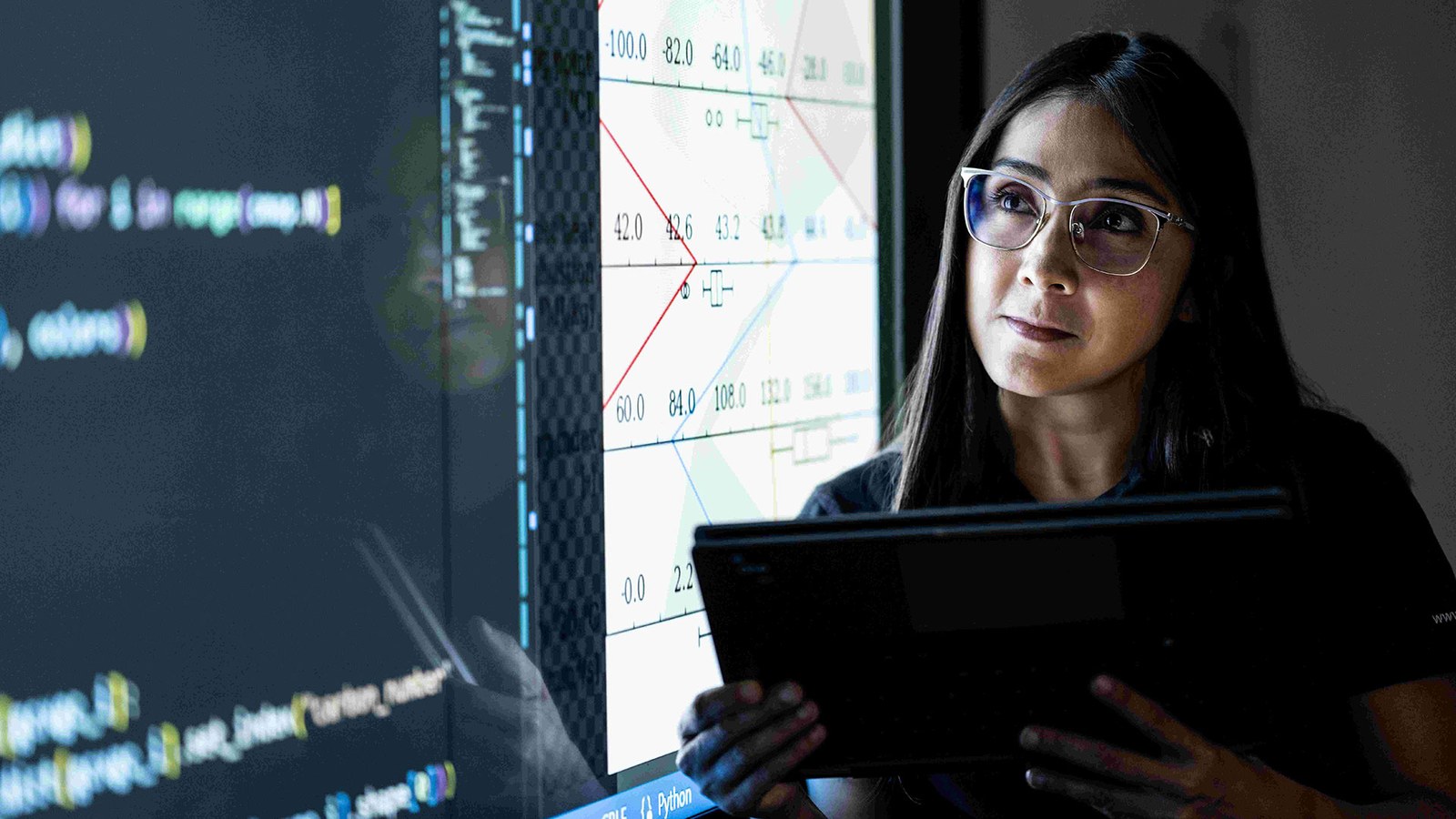
Fuel Impact Assessment
It is important to understand the complex interplay between fuel, aircraft and climate. In this context, we investigate how changes in the composition of aviation fuel can influence several aspects of aircraft performance, including fuel efficiency and emissions of pollutants such as carbon dioxide, nitrogen oxides, and particulate matter. The aim is to maximize the benefits of using sustainable fuels and determine the additional value they contribute in terms of local air quality, environmental sustainability, decreased carbon footprint, and aircraft performance. We accomplish this by using computer models, while also coordinating flight tests specifically tailored to assess emissions and climate impact. For example, we have already shown that the use of sustainable aviation fuels, which are produced from renewable sources such as used cooking oil and agricultural waste, can significantly reduce the climate impact while also improving air quality.
Optimization of Future Refineries
We want to boost the production of climate-optimized fuels by the most efficient production processes and refinery configurations. Therefore, direct and iterative feedback on the production process is provided through application of the prescreening method and tools. This aims especially at the initial phases of production process development, when only limited volumes are available for experimental testing, and fuel producers face a lack of information regarding the suitability of their fuel for approval. Our continuous efforts involve enhancing our fuel design tools by incorporating additional process models to assess the influence of different production steps on fuel quality, beginning with upgrading processes such as hydrotreatment and distillation. With the help of this information, we contribute to the process design of future refinery concepts and offer feedback to guide process design decisions. Our ultimate motivation is to minimize climate impact of aviation by helping to design SAF refineries for optimum SAF through co-optimization of the production process and the fuel.
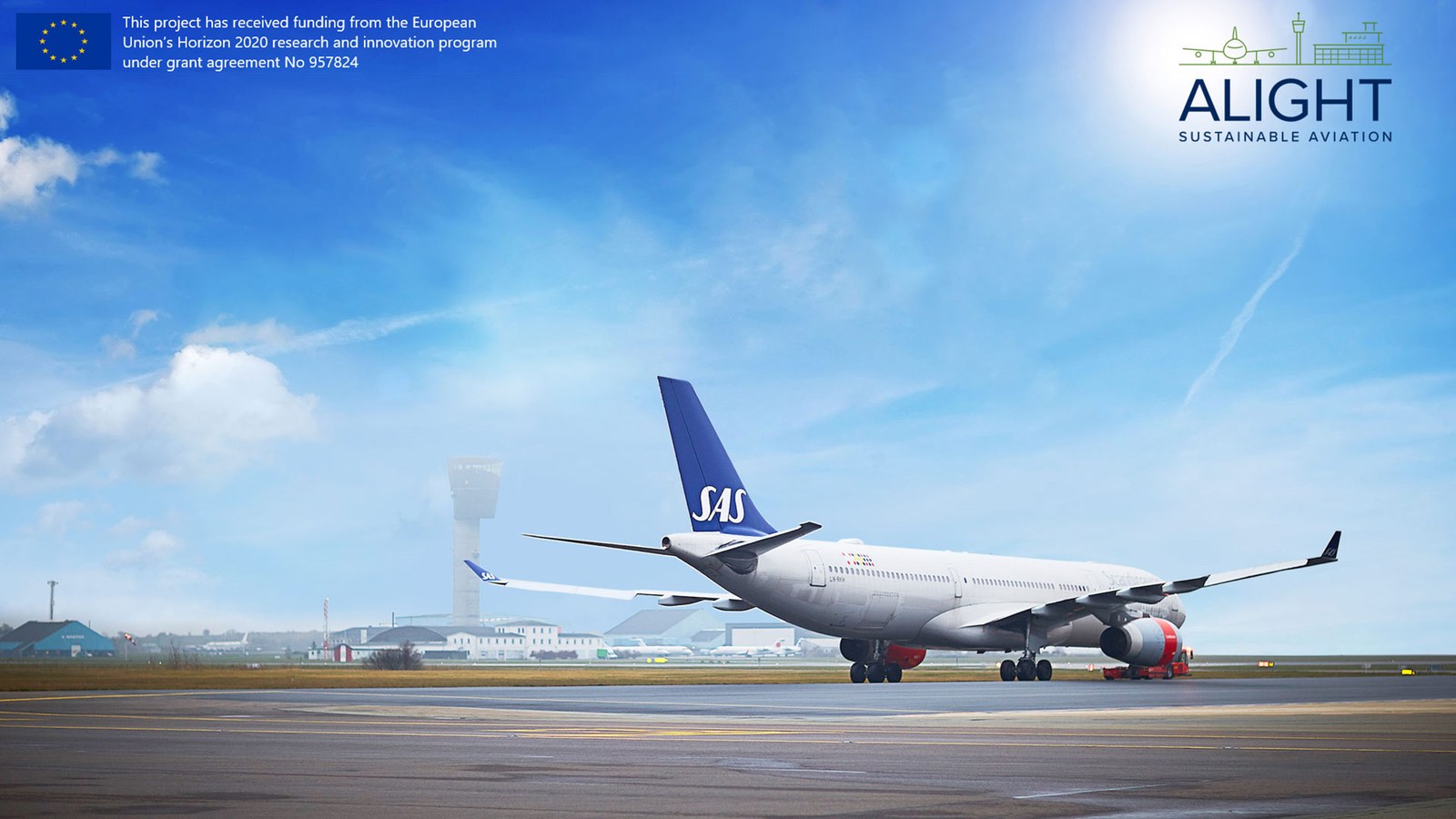
ALIGHT
Smart Use of SAF
We explore possibilities to realize the maximum climate impact reduction of SAF during flight missions. This includes targeting the available amounts of SAF to flights with high climate impact and optimized refueling strategies. For this purpose, information and data must be collected and made available over the entire life cycle of a SAF. As risk-related decisions rely on this information, it is crucial to systematically document and assess uncertainties in both data and models. To facilitate this, a digital thread representing the process and information chain is employed together with digital twins for SAF.
Uncovering specific sub-processes of fuel use and fuel production
The combustion chamber is a fundamental component of gas turbines, which are the most commonly used engines in aviation. An efficient combustion not only leads to lower fuel consumption but also to significant less pollutant emissions. Via multi-phase flow simulations, we study how fuel affects combustion sub-processes like atomization, dispersion, vaporization, chemical reactions, and in the end emissions.
Additionally, we explore cutting-edge technologies in fuel production, such as Direct Air Capture (DAC). Through the use of numerical simulations, our research focuses on the intricate multi-phase sub-processes, striving to propel advancements in DAC systems and improve system design, efficiency, scalability, and cost-effectiveness.