Turning image data into an atlas – Mapping the moons of Saturn

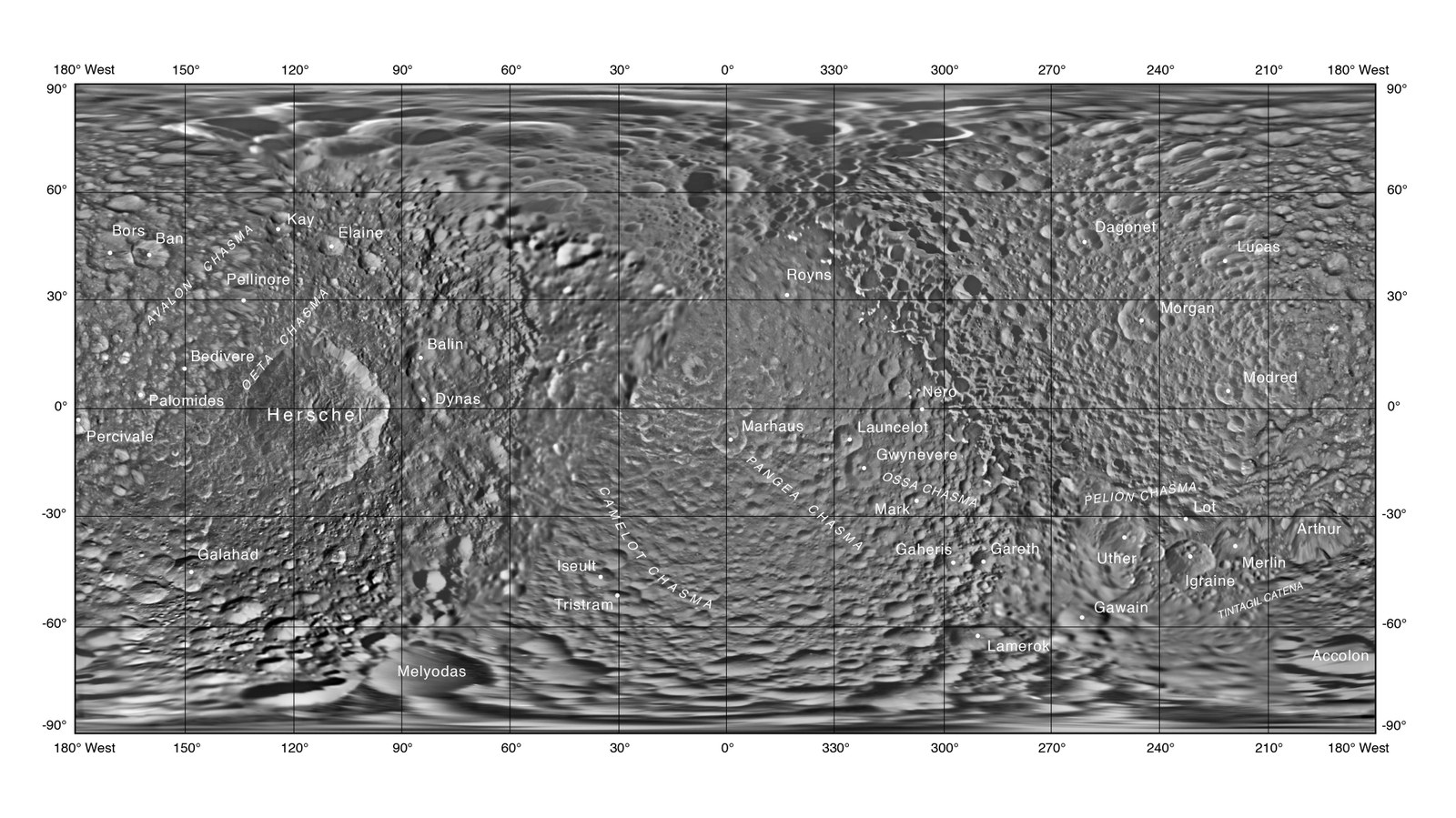
On 15 September 2017, NASA's epic Cassini-Huygens mission will come to an end after 20 years. The German Aerospace Center (Deutsches Zentrum für Luft- und Raumfahrt; DLR) has been involved in the mission from its inception, and continues to be involved both scientifically and technical. The DLR Space Administration has been funding the venture on behalf of the German Federal Ministry for Economic Affairs and Energy (BMWi).
Our series of articles will look back on the mission, its experiments, the scientific concept, its most important findings and the contributions provided by DLR and other scientific institutes in Germany.
Today, thanks to Cassini, we know 62 of Saturn's moons. Maps are important to be able to study them further. The DLR Institute of Planetary Research has produced maps for the seven medium-sized ice moons Mimas, Enceladus, Tethys, Dione, Rhea, Iapetus and Phoebe, based on high-resolution images acquired by the camera on board Cassini. Titan, Saturn's largest moon, has a dense atmosphere that does not allow for high-resolution images in the visible light spectrum to be acquired, so its mapping is based primarily on radar data. The other moons are mainly very small objects with a diameter of less than 100 kilometres that rotate in such an irregular way that it is impossible to pin a coordinates grid on them.
The imaging technology
The Cassini camera system ISS (Imaging Science Subsystem), which was used to produce the images for the maps, consists of two components: the high-resolution camera NAC (Narrow-Angle Camera), which has a telescope with a focal length of two metres, and the wide-angle camera WAC (Wide-Angle Camera) with a focal length of 20 centimetres. Each camera has several filters that together cover a wavelength range from the ultraviolet to the infrared. The panchromatic images and those taken with the green filter are relevant in the production of maps, as they provide approximately the same degree of contrast. This combination achieves the fullest possible coverage at high resolution.
But before these square images – each raw image has a size of 1024 by 1024 pixels – can be assembled to produce a global mosaic, it is first necessary to review all images of a moon and define the resolution required for the mosaic in metres per pixel. This is because, unlike in other missions, Cassini did not orbit the moons until their entire surface was mapped. Instead, its orbital path was around Saturn, and Cassini acquired images of the surface of each moon during every close flyby. Although these imaging sequences were planned precisely, the probe flew over the surface at a different altitude each time. In addition, the Sun was positioned at a different angle above the moons' horizon, and the camera's angle relative to the surface also changed each time. Ultimately, this produced very heterogeneous images and made calculating the mosaic an extraordinarily difficult task.
Processing the raw data
The Cassini spacecraft beamed the images down to Earth in the form of raw data. Scientists back on Earth then assigned information about the position of the orbiter and the precise location at which the camera was directed – known as 'pointing' – to the recorded data based on the positional data that the spacecraft itself recorded at the time of image acquisition. While the orbit information is sufficiently precise to continue processing the images, reconstruction of the camera pointing required some improvement (fig. 2). Images showing the edge of the moons from a significant distance can be corrected in this way, while those with high resolution (close-ups) are adapted with photogrammetric precision by comparing them to the images showing the edges. The received images do not only lack spatial reference, some of them also contain export errors or other image errors (fig. 3a). Once these problems are ironed out, other corrective steps are completed: radiometric calibration (fig. 3b) is used to remove sensor errors – background noise – in the digital data, along with deviations in the data produced by radiation. As the objects on the surface were recorded from a different direction each time, this means that their shadows would point in varying directions. To mitigate the effects this would have on producing accurate maps, photometric corrections are carried out to standardise the position of the Sun in each image at the time of acquisition.
The final stage involves a geometric correction of the images to ensure that all objects are shown correctly relative to the surface and each other and that there is no offset from the edge of one image to the next. The images are then converted into the chosen map projection (fig. 3c) and fitted together to produce a global mosaic.
Division into map sections
The next step is to decide on the number of map sections that the mosaic can produce without losing image resolution, while still producing maps of a manageable size. Here, a standard reference work produced by two US scientists at the end of the eighties defines the benchmark of what planetary atlas sections should look like. Three sectional layouts were defined for Saturn's ice moons over time: Enceladus, Tethys, Dione and Rhea are each spread over 15 maps (fig. 4); Mimas and Iapetus over three; and only Phoebe was reproduced in just one map. Of all the moons listed here, Phoebe is the furthest from Saturn, and was only visited by Cassini once, on 11 June 2004, at the start of the mission.
Once the correct sectional layout has been selected, the mosaic is divided up and converted into the correct map projection. In the next step, a map grid is constructed around each of these mosaic sections. The coordinate system follows that defined by the International Astronomical Union (IAU) with latitudes running westwards and ascending from 0 to 360 degrees. The prime meridians are defined by a small crater on the surface. Additional information is then added to each map sheet, as well as to the main map. This includes a global overview map with information on the location of the map section – an insert with the individual images included in the respective sheet and a chart with their resolutions.
A Thousand and One Nights and King Arthur
Like all celestial bodies in the Solar System, topological features like craters, mountains, planes or rift structures are named after mythology or cultures of the people. They were proposed by the Cassini Imaging Team, which also included DLR scientists and cartographers, reviewed and then confirmed by the IAU. Each moon was assigned a certain theme, from which names of people or places were taken. For instance, the legend of King Arthur was used for Mimas, 'A Thousand and One Nights' for Enceladus, or Homer's Odyssey for Tethys. All names are noted in the map sheets. The Gazetteer of Planetary Nomenclature by the U.S. Geological Survey contains a wide range of categories generally used to name objects on planets, moons and asteroids.
New images continuously improve the maps
The mission yielded a constant stream of new, spectacular images from close lunar flybys between 2004 and 2017. It was therefore possible to continuously update and improve the mosaics and atlases. New regions were illuminated that had never before been seen in such detail. Other structures were discovered that were so prominent and of geological significance for the respective moon that they deserved their own name. All of the atlases and image mosaics are available to the public and can be downloaded from the websites at Cassini Imaging Central Laboratory for Operations or NASA's Planetary Data Systems.